Physicist: This question really appeals to my ten-year-old self. If you’ve never tried to burn something with a lens, collect three pairs of sunglasses, a magnifying lens, and something you dislike. On a bright day, put on all three pairs of sunglasses and give it a shot.
Typically, when you try to focus sunlight with a lens you’ll get something that looks like a comet. You turn the lens and move it up and down and just at the moment when the bright spot gets the smallest, you suddenly see clouds. This is because the smallest you can concentrate light from the Sun using a lens is an in-focus image of the Sun, and incidentally, an in-focus image of anything else in the sky as well.
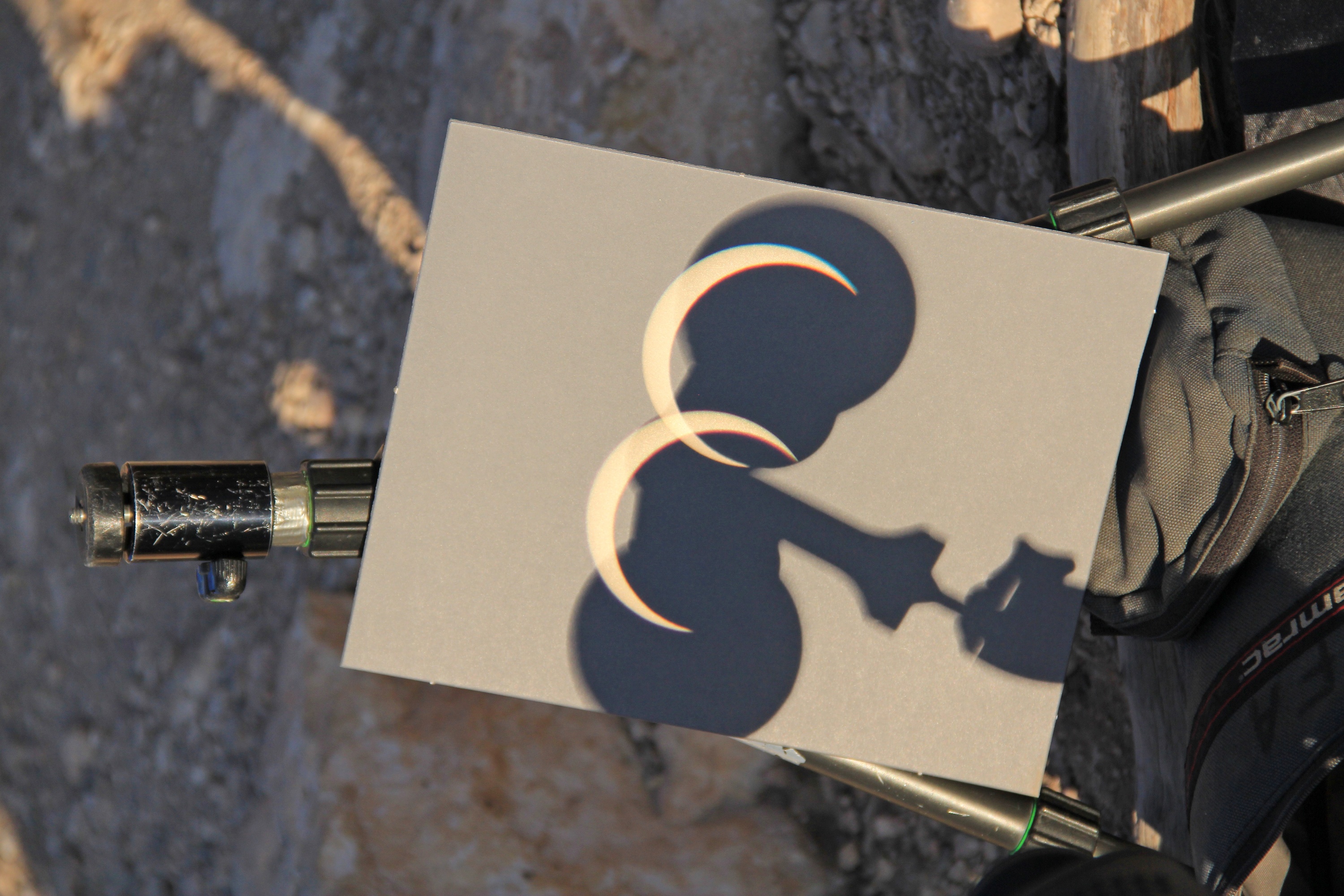
Paper held in the “focal plane” of a pair of binoculars during an eclipse. This is the smallest that the light can be focused. If the paper were any closer or farther away the images of the Sun would become blurry and more spread out.
There are several types of lens, depending on how the two sides are curved, but in each case the defining characteristic is the “focal length”, f. The big rule for lenses is: parallel light collects together at the same place, the “focal point”, which is f away from the lens.
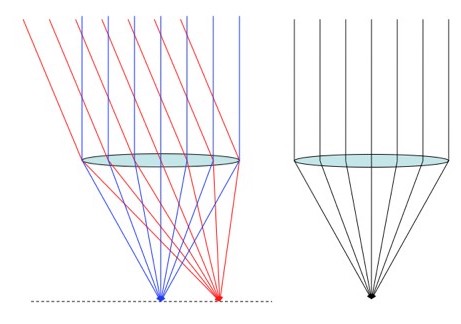
Left: Parallel light beams collect together at a focal point, with a different point for each different set of parallel beams. The collection of these points is the “focal plane”. Right: Here we’ll consider the simplest case, light perpendicular to the lens, since the results apply in general and we can talk about the focal point (“the focus”) instead of the focal plane.
An “image” is the location where the light from an “object” is brought together by a lens. The image is so named because if there happens to be a screen at that location, an image of the object will appear in-focus. The distance to the image depends on the distance to the object.
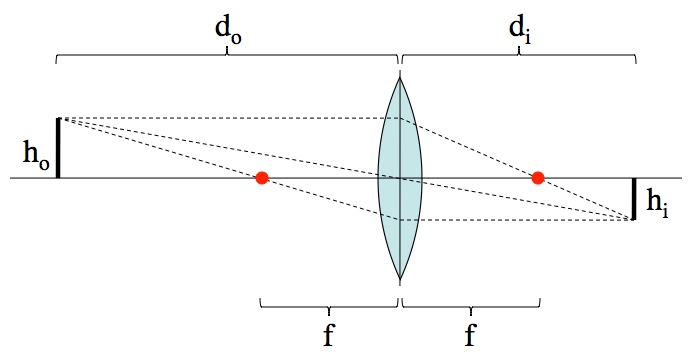
For lenses, the rules are: #1) Parallel light beams will pass through the focus (dots) on the far side of the lens. #2) Light passing through the center of the lens doesn’t change direction. These rules allow us to figure out the size and location of the Image given the size and location of the Object (black bars).
It takes a little algebra (included in the “answer gravy” below), but rule #1 in the caption above leads to the Thin Lens Equation:
where do is distance from lens to object, di is the distance from lens to image, and f is the focal length of the lens.
Rule #2 is easier to work with and, with a little less algebra, leads to the magnification equation:
where hi and ho are the sizes of the image and object. M is the factor for how much bigger the image is than the object. For a microscope, you want M to be big.
The distance to the Sun, do, is infinite in every useful sense. That’s why it took so long to figure out how far away it is. As far as (reasonably sized) lenses go, a few miles may as well be the other side of the universe. The distance to the Sun is somewhere in that “may as well be on the other side of the universe” range. In fact, everything in the sky falls into the same category, which is why the night sky looks like a dome, rather than the unending void that it is.
Plug do= into the thin lens equation and you find that
, and so di=f. In other words, the Sun, and everything else “at infinity”, will be in focus f away from the lens. This coincides with the definition of the focal length, since light from a source at infinity is always parallel. That should jive with your our experience: if you look at a light ten feet away and you step back and forth, the angle to the light changes, but if you look at the Sun (don’t) and step back and forth, the angle to the Sun stays the same.
Now on the face of it, it would seem as though there’s no way to figure out the size of the Sun’s image, hi, since . As with the resolution to so many other unwanted infinities, all we need is a little algebra.
Without some fairly sophisticated techniques, it’s impossible to gauge how far away the Sun is. But while do is out of reach (for most of us), isn’t. By measuring the Sun’s apparent size in the sky, it’s easy to figure out that it’s 110 times farther away than it is big. The same thing, very coincidentally, is true of the Moon; it is 110 Moon-diameters away from Earth. Mathematically speaking,
.
Retentive readers will recall that we haven’t brought the magnification equation, , into play. That was intentional; pretending there’s an issue heightens drama. Solving for the image size, hi, and plugging in what we already know,
and di=f, we get:
So, how big is the bright spot when you focus sunlight? At best, a little less than 1% of the distance to the lens. To concentrate light from the Sun as much as possible, you want to position the target (the solar oven, the ant trail, the piece of wood, whatever) at the lens’ focal distance. When you do, the bright spot will have a diameter of . This ultimately comes down to the fact that the Sun is really far away, and 110 times smaller than it is distant.
The bigger a lens is, the more Sunlight it can gather. So the best lenses for burning stuff are as big as possible (more light), with the shortest possible focal length (tiny spot).
Answer Gravy: Every now and again, it’s worth seeing how statements of fact turn into math. Geometric optics (which sounds much more impressive than it is) basically boils down to the two rules mentioned above:
#1) Parallel light beams will pass through the focus on the far side of the lens.
#2) Light passing through the center of the lens doesn’t change direction.
The thin lens equation almost immediately falls out of these rules and the geometry of similar triangles. Rule #2 is the easiest to work with. Looking at the line that passes through the center of the lens, we find two similar triangle on either side. The triangle on the left has legs ho and do and the other with legs hi and di. Since these triangles are similar, the ratio of these lengths are the same: . Rearranging this to put the h’s and d’s on opposite sides produces the magnification equation,
. Easy!
Using the same basic trick on the triangles formed by rule #1, we can find the thin lens equation. Looking at just the right side (which side doesn’t matter), there are two triangles similar to each other. A smaller one with legs f and ho and a larger one with legs di and ho+hi.
And there it is. We start with a pair of intuitive, but difficult to apply principles, and end up with a pair of unintuitive, but easily applicable equations.
why is this important? for our investigatory project