Physicist: In very short: nope.
The second law of thermodynamics is sometimes (too succinctly) stated as “disorder increases over time”. That statement seems to hold true, what with mountains wearing down, machines breaking, and the inevitable, crushing march of time. But living things seem to be an exception. Plants can turn dirt (disordered) into more plants (order), and on a larger scale life has evolved from individual cells (fairly ordered) to big complicated critters (very ordered).
However, there are a couple things missing from the statement “disorder increases over time”, such as a solid definition of “disorder” (it’s entropy) and the often-dropped stipulation that the second law of thermodynamics only applies to closed systems.
Creatures, both in the context of growing and reproducing, and in the context of evolution are definitely not closed systems. Doing all of that certainly involves an increase in order, but at the expense of a much greater increase in disorder elsewhere. Specifically, we eat food which, with all of its carbohydrates and proteins, is fairly ordered, and produce lots of heat, sweat, and… whatnot. Food, and air, and whatnot are what make living things “open systems”.
If a creature could take, say, a kilogram of non-living, highly disordered material and turn it into a kilogram of highly ordered creature, then that would certainly be a big violation of the second law of thermodynamics. However, people (for example) consume along the lines of about 30 to 50 tons of food during the course of a lifetime. Some of that goes into building a fine and foxy body, but most of it goes into powering that body and fighting degradation (blood and skin and really everything wears out and needs to be replaced). So, about 0.15% (give or take) of that food matter is used to build a body, and 99.85% is used for power and to fight the entropy drop involved in body construction and temporarily holding back the horrifying ravages of time.
When compared to the entropy involved with turning food into the many, many bodies that make up a species, evolution is barely an afterthought. In fact, the entropy (as used/defined in thermodynamics) of most animals (by weight) is all about the same. A person and a mountain lion have about the same entropy as each other, simply because we weigh about the same.
The big exception is photosynthesizing plants. They really can turn a kilogram of inert, high-disorder dirt, air, and water into a kilogram of low-disorder plant matter. But, again, they’re working with a bigger system than just the “plant/dirt/air/water system”.
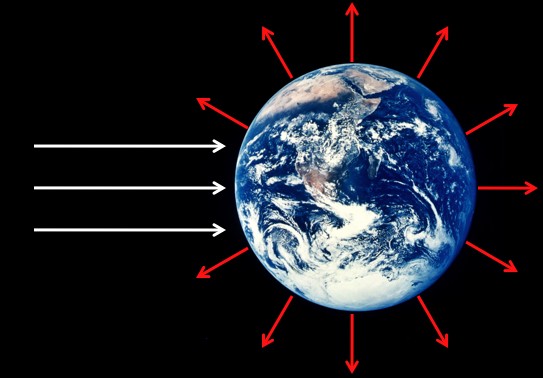
There’s a huge increase in entropy between the incoming sunlight and the outgoing heat that’s radiated away from the Earth.
Sunlight is a bunch of high-energy photons coming from one direction, which involves relatively little entropy. A little later that energy is re-radiated from the Earth as heat, which is the same amount energy spread over substantially more photons and involves a lot more entropy (relatively). This huge increase in entropy, between the incoming sunlight and the outgoing heat, is the “entropy sink” that makes all life on Earth possible (with just a handful of exceptions). In particular, green plants take a tiny amount of the sunlight that hits the Earth and turns some of the energy into sugars and other useful plant-ey material. It all eventually turns into heat and radiates away, but instead of doing it all at once it does it through a few links in the food chain.
You can think of this huge sunlight-to-re-radiated-heat increase in entropy like water going over a waterfall, and life as being like a hydro-electric dam. It all ends up at the bottom of the falls, but sometimes it can do some interesting stuff (life and other useful mechanical work) on the way.